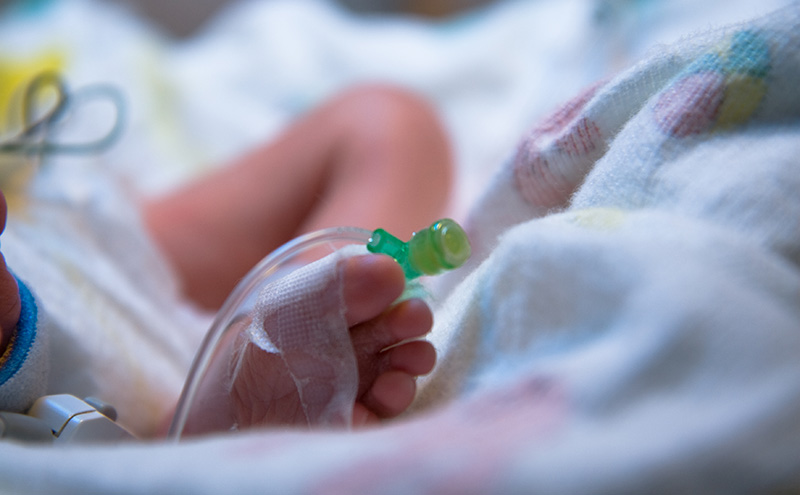
August 30, 2021
Trouble in the Tasman Sea: Pediatric Diabetic Ketoacidosis (DKA)
By Mark Cimino MD, MBA
Twenty-four hours into our two-day crossing from Australia to New Zealand, parents presented to the medical center with their 1-year-old child with complaints of crying, excessive sleeping, not playing, weakness and clinging to his mother more than usual. The mother related that the child had been to the pediatrician four times in the past two weeks before coming on the cruise, with the most recent visit just two days prior to embarkation. The child was treated for tonsillitis and prescribed Augmentin on the first visit. His symptoms resolved, only to return two days before embarkation, and he was given another round of Augmentin. His mother had also reported to the pediatrician that he was drinking a lot more water and urinating more, but no further testing was performed. The pediatrician cleared the child to travel, despite his relapse, repeated antibiotic use and in the absence of a full workup.
On initial observation with the mother holding the child, he appeared withdrawn, pale and uninterested in his surroundings. The mother stated that he had been like this for about 24 hours, just a few hours after they embarked the ship.
On physical examination, the child was in no distress, but appeared tired and was clinging to his mother. He was afebrile, with a heart rate of 134 beats per minute, and he was breathing 20 times per minute. His blood pressure was 104/68. He weighed 11 kilograms. His mucosal surfaces were noticeably dry. His skin was tenting, although his capillary refill did not exceed three seconds. His GCS was 15. He displayed neither lymphadenopathy nor meningeal signs. His affect was depressed, and he appeared generally weak.
During the physical exam of his ears and in proximity to his mouth, the child exuded a very distinct smell of ketones. On history, his mother denied any history of diabetes in the child or in the family members. Our medical staff were now faced with a seriously ill child while at sea, without the option of a medical evacuation for more than 24 hours. His glucose was 657 mg/dl. He was admitted to our ICU, an IV line was placed in his right foot and later the left antecubital vein. Blood gas analysis, urinalysis and a CBC were obtained. During the ensuing hours, this child became the most challenging case to manage on a cruise ship in my 20-year career.
Initial Labs were as follows:
Venous Blood gas | ||||
PH 7.17 (7.31-7.41) | pCO2 32.2 (41-51 torr) | pO2 37 (30-40 torr) | ||
HCO3 11.9 (22-26 mEq/L) | TCO2 13 (20-29 mmol/l) | Base -16 (-2 to +3) | sO2 58 | |
Chemistry (I-stat) | ||||
HCT 42 | HGB 14.3 | Creat 0.2 | Glu 657 | Chloride 104 |
NA 131 | K 4.9 | TCO2 12 | Anion gap 21 | iCa 1.26 |
Corrected Na Na+[(glucose-100)x0.016] = 140 | ||||
Urine dip | ||||
Urine Glu >2000 | Ketones Large PH 6.5 | Nitrate Neg | Leukocytes Neg | |
CBC | ||||
Hematocrit 46.5 | HGB 15.6 | WBC 13.4 | Platelets 392 |
Clinical Course
The child was given an immediate bolus of 250cc NS and his sugar was rechecked. The bolus lowered the glucose by 150 mg/dl, to 507 mg/dl. However, his heart rate was not improved by the saline bolus, and he was thus provided with another 100ml bolus over 30 minutes. On repeat following this second bolus, his glucose was 397 mg/dl. He was started on oxygen at 2 liters by nasal cannula, as his pH was 7.1. Pulse oximetry cannot be fully relied upon, based on the pH oxygen disassociation curve, when tissues are inherently hypoxic despite good perfusion.
To limit lab errors, fluids were infused through the intravenous line placed in the child’s foot, while the antecubital vein was used for drawing blood. After the second bolus, he was started on 1 unit of regular insulin per hour, and fluids were changed to lactated Ringer’s solution and infused at 30ml per hour. A two-bag system was utilized. The second blood draw produced and dramatic drop in potassium. The bag of lactated Ringer’s solution was replaced with a new bag of lactated Ringer’s solution with 20meq of potassium and 75mmol of 50% dextrose to make a D5% lactated Ringer’s solution. This was done to combat the quickly falling sugar and to ultimately maintain a glucose level of approximately 150mg/dl while continuing fluids to close the anion gap.
Blood analysis between the second and third draw became most concerning. His pH and Co2 had worsened, his blood pressure suddenly dropped to 93/43 and he became more lethargic. Cerebral edema was considered and was concerning since we did not stock Mannitol. The insulin infusion was stopped briefly and another bolus of 50cc of lactated Ringer’s solution was given. His blood pressure responded to the bolus and the insulin was reduced to 0.5mg per hour and the fluids were increased to 50ml per hour in an effort to increase the potassium level, slow the potassium migration back into the cells, and maintain his blood pressure.
Over the course of the 24-hour treatment, we continually adjusted the fluid infusions and the insulin dosage given. Our main goals were to try to maintain his blood pressure, maintain a glucose around 150 mg/dl, arrest the falling potassium to prevent cardiac arrhythmias and slowly increase the pH and close the Anion gap, all while trying to prevent cerebral edema.
Diabetic ketoacidosis (DKA) is biochemically defined as a venous pH less than 7.3, or a serum bicarbonate concentration of less than 15 mmol/L, a serum glucose concentration greater than 200mg/dL in the presence of ketonemia, glucosuria, and ketonuria. The severity of DKA is determined by the degree of acidosis. DKA is the result of a critical relative or absolute deficit of insulin, resulting in intracellular starvation of insulin-dependent tissues, stimulating the release of the counter-regulatory hormones such as growth hormones, cortisol, catecholamines and glucagon. These hormones stimulate lipolysis and proteolysis, hepatic and renal glucose production, and hepatic oxidation of fatty acid to ketone bodies. Unlike during physiologic fasting, absence of citric acid cycle processing of the glucose impedes the processing of these ketones for energy. The frequency of new-onset diabetes presenting as DKA varies widely by geographic region. As was the case with this child, previously undiagnosed patients in DKA have been recently seen in physicians’ offices or emergency departments without adequate history and laboratory studies that could have revealed the diagnosis before the onset of critically illness.
Our initial goals of treatment were to restore perfusion, increase glucose uptake, increase glomerular filtration, and reverse progressive acidosis. We arrested ketogenesis with insulin administration. Dehydration was addressed before insulin was started, providing a more realistic starting glucose level. To prevent rapid declines in plasma glucose, a two-bag system is recommended with fluids and insulin separated and potassium replacement can be provided as half potassium phosphate (KPO4) and half potassium acetate; which like lactate, is converted to bicarbonate to help correct acidosis. Bicarbonate administration is not indicated in pediatric DKA. If blood glucose falls below 150 mg/DL, a higher concentration of sugar must be provided and the insulin dose reduced. Potassium must be closely monitored, as it can rapidly fall, causing arrhythmias. Insulin should not be stopped, and a continuous supply is needed to prevent ketosis and permit continued anabolism. Persistent acidosis after 8 to 10 hours of treatment is usually caused by inadequate insulin effect and could be due to the insulin dilution or rate of administration. Too dilute a solution may enhance insulin adherence to the tubing. It should be noted that central venous catheter placement in children may be associated with deep vein thrombosis and is not recommended in the pediatric population.
Most diabetes-related morbidity in childhood can be attributed to complications of DKA. Cerebral edema accounts for 60% to 100% of this mortality. Other causes of death or disability with DKA include hypokalemia, hypophosphatemia, hypoglycemia and other intracerebral complications. Hypophosphatemia can cause progressive muscle weakness and death due to cardiorespiratory arrest days after metabolic recovery from DKA. Administration of half of the potassium as KPO4 may prevent this. To replace potassium with solely KPO4 can result in hypocalcemia.
Our pediatric patient improved over a 24-hour period under our care and the ship was diverted to a port that provided a pediatric intensive care physician. I accompanied the child and his mother to the hospital and supplied a direct hand-over report to the receiving doctor. After 14 days in the ICU, he was transferred back to Australia by air ambulance. Fortunately, because appropriate care was available at the receiving facility, and our medical center staff did not feel it necessary to return him to our care onboard.
Shipboard physicians must be diligent about where we conduct disembarkations of sick individuals. Requesting a helicopter evacuation from a ship at sea is not without risk, and we, at times, actually may be doing greater harm to a patient or our crewmembers by requesting such disembarkations. The ship must be correctly positioned to maintain speed while the helicopter hovers above the deck, creating a 200-mile-per-hour downdraft. The noise is so loud that staff must communicate by hand signals. The patient must be secured, and we must be absolutely sure that any sheets are secured as these can fly into the rotors and cause the helicopter to crash.
Shoreside physicians are often asked to provide medical clearances for their patients prior to a voyage. A better understanding of the medical capabilities along a ship’s itinerary, the limitations imposed by evacuation procedures and an appreciation of the limitations to shipboard medical care would go far in helping optimize these decisions. In the case of our pediatric patient, a more thorough workup ashore might have averted a serious medical crisis at sea.